Seven-Foot Cones Key in New Approach to Nuclear Energy
By Chris Bryant and Mary Wymer
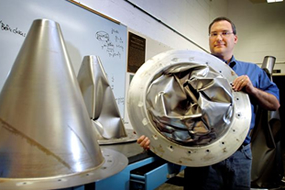
From national security issues, to high oil prices, to environmental impacts, reasons for the growing search for alternative energy sources are broad-based. Now, a national security laboratory, with an assist from a University of Alabama engineer, is using what appear to be giant, steel traffic cones to try and turn nuclear fusion into a realistic energy alternative.
Dr. Mark Barkey, a professor of aerospace engineering and mechanics, was awarded a $158,000 grant from Sandia National Laboratories, a multi-program national security lab, to test a key element of a new base for developing a nuclear power plant.
Today’s nuclear power plants are based on fission, but Sandia is interested in developing the different process, nuclear fusion. As its power source, nuclear fusion uses forms, or isotopes, of hydrogen called deuterium and tritium. Producing energy using such readily available sources is appealing.

The overgrown cones are key. Some 7 feet tall, but only about thirty-thousandths of an inch thick, the cones, technically known as Recyclable Transmission Lines, are nested, one inside another.
The cones are used to deliver an intense pulse of energy to a tungsten wire array surrounding a fusion target containing the hydrogen isotopes. This delivery to the wire array produces intense X-rays that heat and compress the fusion target to the energies and densities required for fusion. This fusion reaction generates energy that eventually could be harnessed to produce electricity. The nested cones must maintain a high vacuum between them without deforming for this concept to work.
The main distinction between Sandia’s vision of nuclear fusion and other proposed forms of generating power from nuclear fusion is that Sandia’s proposal relies on pulsed power, or repeated fusion reactions, as opposed to attempting to create a continuous fusion reaction. “Instead of a continuous reaction, it’s like a piston in a car, boom, boom, boom,” Barkey says. These cones are basically the electrodes that would initiate that fusion reaction.”

The cones melt during the process, and with these energy producing blasts needing to be repeated indefinitely to sustain a nuclear power plant, Sandia needs a cost-effective way of producing the cones.
“They are going to make them out of steel and then recycle them,” says Barkey, the UA engineering professor. “The catch is that the reaction will take place every 10 seconds — and these steel cones are partially melted or destroyed each time. When you start adding up all that steel, it gets expensive, so they want to make them as thin as possible. That’s where the engineering comes in. How thin can you make the cones to keep them from buckling — because there is actually a differential vacuum at work on the cones. There is more pressure on the outside of the cones than the inside.”
Barkey suggests thinking of a more familiar object as an example.
“Imagine what would happen if you put more air pressure on the outside of an empty soda can than on the inside,” Barkey says. “The can would collapse just as if you pinched it with your fingers. The same happens with these large cones if the pressure is too great. If the RTL cones collapse, the fusion reaction will not take place.”
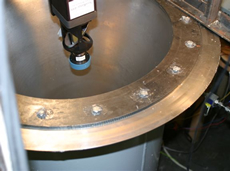
Barkey performs tests on the cones to validate computer predictions of the buckling pressure and to determine what effect initial structural defects have on the critical buckling pressure. He also looked at the impact of placing external stiffening rings around the cone in an attempt to sustain the pressure.
If a fusion-based nuclear plant becomes a reality, it will have a key advantage over traditional nuclear plants, the UA engineering professor says.
The byproducts of fission are a mix of long-lived actinides and fission products. With fusion, the byproduct is just helium and more fuel for the fusion reaction. There are structural materials that become radioactivated with fusion just like with fission, but fusion eliminates long-lived fission products and actinide wastes.
“The work that Dr. Barkey is performing is critical to our understanding of the natural buckling response inherent with these steel walled RTL units,” said Matt Turgeon, senior member of the technical staff at SNL. “The ability of UA to incorporate these large scale tests with rapid turnaround is key to our project, and we hope to continue this relationship with the University.”
Further Reading