UA Physicist’s Predictions Proven True, Leads Industry in New Direction in Quest for Superior Computer Memory
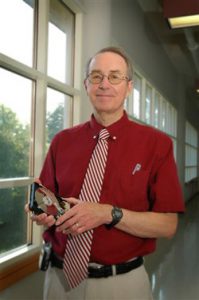
There are no crystal balls visible upon entering Dr. William “Bill” Butler’s University of Alabama office. Yet, theoretical predictions this physicist made in a scientific paper published in 2001 have been verified experimentally and may be key in development of the next generation of computer memory and hard drives.
Butler directs UA’s Center for Materials for Information Technology, or MINT, a multidisciplinary research program focusing on magnetic information storage, including data storage within computers.
The 1990s were probably the golden years of magnetic information storage technology. Every year computer users could, for the same price, purchase a hard disk drive with double the capacity of the previous year. In the past few years, however, this unprecedented technological growth has slowed as the information storage industry has had to face new scientific and technological challenges. Butler’s work, however, (which was performed in collaboration with colleagues Xiaoguang Zhang and Thomas Schulthess at Oak Ridge National Laboratory and James MacLaren at Tulane University) may help the industry achieve a higher technological trajectory.
The rapid development of computer hard drives in the 1990s was enabled by the discovery in 1988 of a new effect called Giant Magnetoresistance or GMR. The first in a series of revolutionary new “spintronic” devices, the GMR effect is used in almost all disk drives sold today.

Spintronic devices are similar to electronic devices but unlike simple electronic devices such as the transistor, they utilize not only the electronic charge but also another property originating in the quantum nature of the electron called “spin”. GMR allowed engineers to make sensors that could sense or “read” extremely tiny magnetized regions on a hard drive. This allowed the manufacturers to pack many more bits of information into the same area. The result, of course, is well known – cheap hard drives, Ipods, TiVos, high capacity memory for digital cameras, etc.
In 1995 scientists started working on a new spintronic effect called tunneling magnetoresistance or TMR that is beginning to appear in hard drives. TMR allows even more sensitive sensors than GMR. A GMR sensor may give a 10 percent change in resistance when a magnetic field is applied. By 2004, scientists had learned how to get a 70 percent effect from TMR.
Then in late 2004, two papers were published that caused tremendous excitement in the magnetic information storage industry. Stuart Parkin at IBM and Shinji Yuasa at Japan’s National Institute of Advanced Industrial Science and Technology announced they could make TMR devices that showed a 200 percent change in the resistance. Since then, reports of changes as high as 260 percent have been published and even much higher values have been achieved. In addition, these new devices have several other helpful properties that may make it possible to incorporate them into commercial spintronic devices.
These new discoveries were not accidental. They were the result of very careful experiments aimed at verifying theoretical predictions made by Butler and his colleagues in 2001. These predictions included several new physical phenomena, which have now been verified. In addition to better, smaller and cheaper hard drives, this new TMR effect may allow the development of a new type of magnetic memory which has no moving parts. This memory, called MRAM, for magnetic random access memory, could, in principle, replace both the RAM and the hard drive because as it can be as fast as RAM yet is non-volatile like the hard drive.
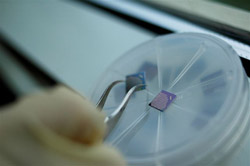
Although MRAM has been under serious development since 1995, the paper, published in Physical Review, shifted the industry researchers’ focus to a different avenue by which they hope to take the budding technology from the laboratory to the user’s desktop.
“There’s hope that magnetic random access memory might be a replacement for the DRAM you now use in your computer,” Butler said. As every computer user experiences daily with DRAM, or dynamic random access memory, an extensive boot-up process is required and power outages can result in lost data. Not so with MRAM, Butler said.
“You could just turn your laptop or hard drive on, and it would be on immediately,” Butler said. “It wouldn’t have the boot-up process, and it would be better for power management as it would need less.” The power savings could greatly increase battery life for laptop and other portable computing devices.
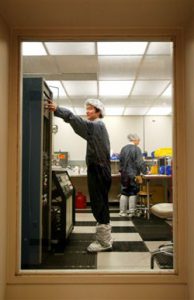
“DRAM has to be refreshed,” Butler said. “That’s why you have to keep it plugged in, but MRAM doesn’t have to be refreshed. It’s what’s called non-volatile memory. When the electricity goes off, it ‘remembers’ the state it was in. In principle, it should be as cheap as DRAM, equal in density, equal in speed, and it should be nonvolatile.”
Flash memory, such as is used to store digital camera images, is also nonvolatile, but saving data to it is slow and cannot be done many times before errors appear, making it impractical for computer memory.
“MRAM might replace DRAM and flash, and it might even possibly replace your hard drive. It could be sort of a universal memory. The military has lots of interest in nonvolatile, sturdy memory,” Butler said.
Despite the high hopes and extreme amount of attention MRAM is getting from developers, it’s not yet on the market. “MRAM is a technology that has been promised to be coming out ‘this year’ for the last several years,” Butler said. “Lots of companies are working on it, lots of companies are making devices, but there is not a product you can buy.”
If a computer company website one day touts its latest machine as “equipped with MRAM,” a key hurdle in the development will likely be traced back to Butler and his colleagues’ 2001 calculations.
As lead author of the paper, Butler performed computer calculations for a previously unknown electronic structure that incorporated multi atom-sized layers of iron and magnesium oxide, with an insulating barrier in between. They predicted the materials’ behaviors under certain achievable conditions.
“We discovered some very interesting new phenomena. This is completely different from the theory people had been using to apply to these types of material combinations, previously.”
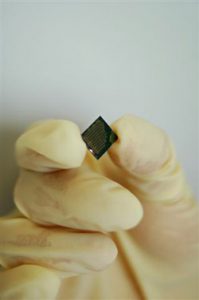
The paper predicted the level of electrical resistance – key in obtaining necessary operating current – in the theoretical electronic structure would be much lower than previously expected. Butler and his colleagues predicted how the TMR values – which enable electrons to occasionally defy the law of conservation and energy – would be much higher than expected. Such high TMR values result in lower resistance which translates into larger voltages. Larger voltage capabilities mean smaller devices can be fabricated with appropriate operating current. And, if the computer industry needs anything, it needs small.
Initially, the reaction to the group’s theoretical calculations was subdued, Butler said. “People had a hard time believing we had done it right because they couldn’t believe the TMR was as high and resistivity was as low as we predicted.”
Theoretical physicists, such as Butler, typically use their calculations to try and explain experiment results. “This is one of the rather few times that a prediction was later observed.”
During the last three major conference of the information storage field, in Jacksonville, Los Angeles and Tokyo, Butler’s predictions and their verification were featured topics.
According to Butler, “this work is just one of many projects at MINT that are transforming the way we store information. This is one of the most exciting areas of science and engineering because you can do fundamental science and yet see important applications. That is really neat. Another neat thing is the way faculty from so many different disciplines have learned to work together.”
Since 2001 Butler has directed MINT, deemed by the National Science Foundation as one of the 29 Materials Research Science and Engineering Centers in the United States. MINT has continuously carried this designation since it first achieved it in 1994 as one of the 11 centers so recognized in the agency’s original selection.
Further Reading
- UA’s Center for Materials for Information Technology
- MRAM-Info: Magnetic RAM news, forums, articles, and more
Contact
Dana Lewis or Linda Hill, UA Media Relations, 205/348-8325, lhill@ur.ua.edu
Source
Dr. Beverly Hawk, Crossroads Community Center director, 205/348-6930, beverly.hawk@ua.edu Brice Miller, Crossroads assistant director, 205/348-8514, brice.miller@ua.edu